So, the short answer is yes. A useful model, at a certain scale.
How can that be? The cosmos is full of stuff. Vast collections of stars, planets, rocks, dust, gas. Chunked over vast distances. Not what our everyday experience considers fluid stuff.
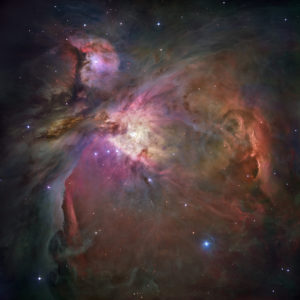
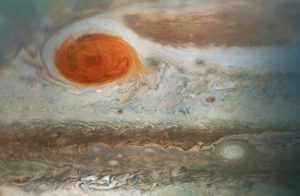
Well, in physics and engineering, fluid dynamics embraces what typically are referred to as liquids and gases (including plasmas).
Wiki: Four states of matter are observable in everyday life: solid [fixed volume and shape], liquid [fixed volume, but a variable shape], gas [variable volume and shape], and plasma [free atoms, ions, and electrons].
Wiki: The solution to a fluid dynamics problem typically involves the calculation of various properties of the fluid, such as flow velocity, pressure, density, and temperature, as functions of space and time.
And as water, for example, is composed of molecules which are composed of atoms which are made of objects which are the subject of particle physics, then a perspective of hard stuff interspaced with vacancy – “solid” and space – fades into one of less and less solid and more and more flow, interactions between flows.[1]
For a huge container of marbles, for example, how might a model of pouring from that container to another be mathematically visualized? What if sand grains fill the container? Or replace the sand grains with H2O molecules – the container is filled with water.
This post was inspired by the teaser for an article titled “Why the universe can be described by the equations of fluids.” Also, that here and there I’ve noted the role of fluid dynamics in my approach to quantum physics – as contoured energy density.
• New Scientist > “Why the universe can be described by the equations of fluids” by Chanda Prescod-Weinstein (3 February 2021) – Studying the universe and the flow of fluids may seem worlds apart, but they involve some of the same equations … (full article requires subscription).
So, I reviewed some other articles on the topic: fundamentals of the standard cosmological model.[2]
• Wiki > “Equation of state (cosmology)“
In cosmology, the equation of state of a perfect fluid is characterized by a dimensionless number w, equal to the ratio of its pressure p to its energy density ρ [rho]. It is closely related to the thermodynamic equation of state and ideal gas law.
• Wiki > “Friedmann equations“
The Friedmann equations are a set of equations in physical cosmology that govern the expansion of space in homogeneous and isotropic models of the universe within the context of general relativity. They were first derived by Alexander Friedmann in 1922 from Einstein’s field equations of gravitation for the Friedmann–Lemaître–Robertson–Walker metric and a perfect fluid with a given mass density ρ [rho] and pressure p. The equations for negative spatial curvature were given by Friedmann in 1924.
Much on this topic reveals how physicists think, build mathematical models using assumptions and heuristics. Models which simplify things (e.g., a “simplified description for matter”) – constrain things; but which might yield useful predictions. Especially as better and more powerful telescopes refine observations. And as computing power enables more and more complex simulations.
Hopefully there’s something like this framework for quantum physics. Especially as the cosmological model depends on models of that “fabric.”
Notes
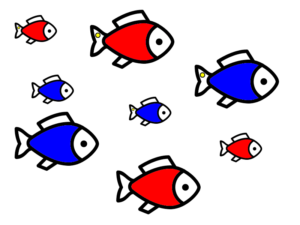
[1] Much like Wilczek’s analogy of us as fish, even our own solidity is a collective flow through occupied spacetime, oblivious to other than (emergent) friction or viscosity between chunks at everyday scales.
“It’s as if we’re very intelligent fish, or super-dolphins, who have figured out after careful scientific study that we are not living in empty space but that we live in water,” Wilczek said during a book-tour stopover in Seattle. “We’re used to it, but we should understand this material – and we haven’t yet figured out what this material is made of.”
See also this comment re the fish parable.
[2] Here’s an example of a technical lecture (2003) on the topic, characterization of the “cosmological energy budget.”
• Assumptions (and evidence) of homogeneity and isotropy for spatial profile (energy-momentum distribution) and the “types of energy in the universe.”
Cosmology as the application of general relativity (GR) to the entire universe would seem a hopeless endeavor were it not for a remarkable fact – the universe is spatially homogeneous and isotropic on the largest scales.
• Einstein’s equation for time evolution of energy-momentum (tensor).
• Selection of a simplifying model for energy-momentum dynamic (tensor).
• Selection of inertial frame of reference.
• Derivation of the Friedmann equation and the evolution equation.
• Selection of local geometry related to the total energy density in the universe (particularly based on measurements of the cosmic microwave background).
• TASI LECTURES: INTRODUCTION TO COSMOLOGY by Mark Trodden and Sean M. Carroll (then at University of Chicago) > Fundamentals of the Standard Cosmology
Before diving right in, it is useful to consider the types of energy-momentum tensors T-sub(µ,nu) we will typically encounter in cosmology. For simplicity, and because it is consistent with much we have observed about the universe, it is often useful to adopt the perfect fluid form for the energy-momentum tensor of cosmological matter.
Note that there is no notion of conservation of “total energy,” as energy can be interchanged between matter and the spacetime geometry.
Within the fluid approximation used here, we may assume that the pressure is a single-valued function of the energy density … [as to “useful cosmological matter sources”].
A constant w leads to a great simplification in solving our equations.
…. it is equivalent to treat any cosmological constant as a component of the energy density in the universe [as a perfect fluid]. … this energy is constant throughout spacetime; we say that the cosmological constant is equivalent to vacuum energy.
Meditate on this visualization of swirling liguids.
• Colossal > Art > “Paint, Oil, Milk, and Honey Mix in this Surreal Macro Video of Swirling Liquids by Thomas Blanchard” (August 12, 2015) – Vimeo video.
Patterns of turbulence all the way down …
Credit: Thomas Blanchard
Notes
• Quanta Magazine > Fluid Dynamics > “Mathematicians Prove Universal Law of Turbulence” by Kevin Hartnett (February 4, 2020)
• Wiki: Batchelor scale
Batchelor’s law. Phenomena inside phenomena.
Super-liquids (or super-fluids) are active subjects of research in quantum physics. Theories and models. At cosmic and microscopic scales.
At cosmic scales, modeling the universe as a perfect fluid is a useful model.
On the opposite end of the powers of 10 scale, there’s quark-gluon plasma (QGP), a state of matter (quark matter) which may be modeled as a perfect fluid as well [1].
Wiki:
It’s interesting that fluid dynamics – at least as part of superfluid theories – may be used in practical models at a range of scales. From the pre-matter state of the primordial universe; quark matter in black holes and in some types of collapsed stars; and generally in “melted” hadrons (and perhaps even proton soup).
And perhaps the quantum vacuum (aka space-time) itself, as in Wilczek’s Grid, regarding quark-antiquark background [2].
Wiki: “Superfluid vacuum theory (SVT) … [might] describe all known interactions [colloquially forces] and elementary particles as different manifestations of the same entity, superfluid vacuum.”
Notes
[1] Re research from Big Bang to particle accelerator bangs …
Regarding the Big Bang and quark-gluon fluid, this article presents an overview of research at the LHC exploring primordial matter.
• Earth Sky > “The 1st Microsecond Of The Big Bang” by Deborah Byrd (June 16, 2021) – The peer-reviewed journal Physics Letters B has published this new work online for its July 10, 2021, issue.
[2] Re quantum vacuum energy > Comment June 30, 2019:
So, more research on quark-gluon plasma as a “perfect fluid” – a subatomic soup.
Interesting because I’ve been wondering about fluid dynamics as applied to exotic plasmas and a possible connection to diffusion equations.[1] Plasma –> “matter” transition.
Also, the fascinating collaboration between the Lawrence Berkeley National Laboratory (Berkeley Lab), the Brookhaven National Laboratory’s Relativistic Heavy Ion Collider (RHIC), and the Large Hadron Collider (LHC) at CERN.
This article includes a 2010 YouTube video which “describes collisions of heavy particles at Brookhaven National Laboratory’s Relativistic Heavy Ion Collider. In 2005, RHIC physicists announced that matter created in the accelerator’s most energetic collisions behaves like a nearly perfect liquid. … the quark-gluon plasma …
• SciTechDaily > “Solving a Cosmic Conundrum: ‘Perfect Fluid’ Brings Us Closer to Understanding How Our Universe Began” by Brookhaven National Laboratory (Oct 1, 2021)
Notes
[1] In my January 7, 2020, comment for my post “Quantum reality, quantum worlds – new book explores quantum foundations,” I remarked:
For image re quantum mechanics and fluid dynamics … While this article is not about quantum field-vacuum interactions, it has an interesting image à la fluid dynamics.
• Phys.org > “Never-before-seen electron behavior could help scientists create superwires for supercharged technology” by Caitlin McDermott-Murphy, Harvard University (December 3, 2021)