[“Communicating science” series] [“Quantum foundations” series] [“What the heck” series]
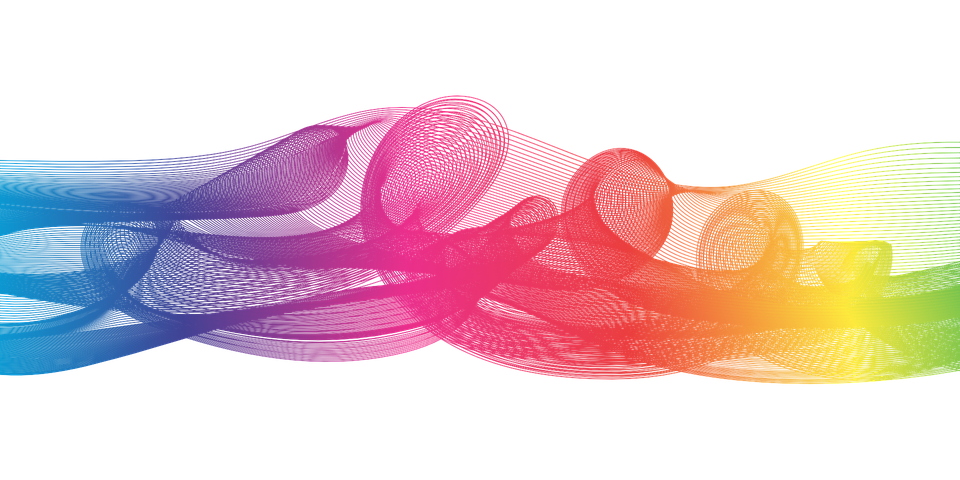
I’ve written about entanglement in prior posts and comments. Over a year ago, I also wrote some preliminary notes. A recent issue of The Caltech Weekly (April 14, 2022) prompted this post. In part because of my ongoing search for better, more compelling analogies (for mere mortals).
The key term (and related concept) is correlation. At the quantum level. So, everyday (macroscopic) objects are ruled out (typically).
And my best sense of this “weird phenomena” entails quantum fields. Pairing of localized excitations in quantum fields. Like in the photon (aka electromagnetic) field. Or the electron / positron field. And persistence of correlation between (relevant) wave function properties.
But how to visualize that? [1]
Wiki:
Quantum entanglement is a physical phenomenon that occurs when a group of particles are:
generated,
interact, or
share spatial proximity
in a way such that the quantum state of each particle of the group cannot be described independently of the state of the others, including when the particles are separated by a large distance.
The topic of quantum entanglement is at the heart of the disparity between classical and quantum physics: entanglement is a primary feature of quantum mechanics lacking in classical mechanics.
An entangled pair
Unpacking quotes and metaphors from the video and article (referenced below), the easiest context for me to understand is where two photons, for example, share a common origin – are created (at essentially the same time) by an interaction between an inciting (pump) photon (from a laser) and a special type of crystal.
From that crystal are emitted two photons (of somewhat lower energy than the inciting one) which share the same quantum state [2], and can be described by a single wave function (by analogy, the same “memory”). A wave function which represents both localized excitations in the photon field – “you have to treat them like a single object.” [3]
[Wilczek] … in all known cases the correlations between an EPR pair must be imprinted when its members are close together, though of course they can survive subsequent separation, as though they had memories. [1]
The relationship between this pair of photons is described as entanglement, an emergent property of the pair (vs. an individual property of either one) – “the most fundamental form of correlations in the world.”
Those excitations propagate in a deterministic manner (according to the wave function). In a smooth (mathematically differentiable) landscape. So, these photons’ quantum properties are duly correlated (entangled) until altered (disturbed) by future interaction(s) with other field excitations (or perhaps with the quantum vacuum).
While entangled, when a relevant quantum property of one of those photons is “measured” later at some point in space and time, the same property of its “twin” also is known.
Returning to our dancer metaphor, this would be like observing one dancer and finding them in a pirouette, and then automatically knowing the other dancer must also be performing a pirouette. The beauty of entanglement is that just knowing the state of one particle automatically tells you something about its companion, even when they are far apart.
The article contains a helpful analogy using macroscopic balls which can be measured (observed) as either yellow or red. The key concept is superposition: “the entangled information about color does not lie within any one ball but exists in the connection [relationship] between the balls.”
An entangled congregation – many-body entangled systems
Entanglement can also occur among hundreds, millions, and even more particles. The phenomenon is thought to take place throughout nature, among the atoms and molecules in living species and within metals and other materials. When hundreds of particles become entangled, they still act as one unified object. Like a flock of birds, the particles become a whole entity unto itself without being in direct contact with one another.
My take
While “today’s quantum scientists say there is nothing spooky about it,” entanglement remains bizarre.
Thanks to theoretical work by John Stewart Bell in the 1960s, and experimental work done by Caltech alumnus John Clauser (BS ’64) and others beginning in the 1970s, scientists have ruled out these local hidden-variable theories.
“There can be correlation without communication,” and the particles “can be thought of as one object.” [Thomas Vidick, Caltech professor of computing and mathematical sciences]
It’s the distance thing – over vast (arbitrary) separations (in spacetime). And speculation that “the particles really [are] somehow tethered to each other across space.”
Within the mathematical model of quantum field theory, if wave functions are real, can they span arbitrary spacetime separations. Well, the wavelength of extremely low frequency (ELF) photons is in the range of megameters (millions of meters).
So, it makes sense that the wave function of an entangled pair of photons – “as one unified object” – might span arbitrary spacetime separations. But how might that be visualized as field excitation? Are extra dimensions required? Does spacetime itself emerge from entanglement?
Quantum trajectories are so easily disrupted in our everyday turbulent (macroscopic) world. Then measuring one no longer measures all.
Notes
[1] Compare this 2016 article by Frank Wilczek. He discusses the notion of entanglement as: partial knowledge of the state of two systems; and the degree to which knowlege [e.g., measurement] of the state of one system provides [reveals] useful information about the state of the other; and the resulting degree of associated confidence. That extent includes the interplay with quantum “complementarity.”
• Quanta Magazine > “Entanglement Made Simple” by Frank Wilczek (April 28, 2016)
… entanglement between quantum systems arises naturally — for example, in the aftermath of particle collisions. In practice, unentangled (independent) states are rare exceptions, for whenever systems interact, the interaction creates correlations between them. … In quantum theory, states are described by mathematical objects called wave functions.
He uses an example of two simple systems: each “c-on” can be in a round or square state – a single property. The wave functions of the overall states are:
Independent: Φ■ ψ■ + Φ■ ψ● + Φ● ψ■ + Φ● ψ● = (Φ■ + Φ●)(ψ■ + ψ●)
Entangled: Φ■ ψ■ + Φ● ψ●
He then expands the example by adding a second property – imagining that each of the two shapes (circle and square) can also exhibit two colors — red and blue.
Therefore, when measuring a property, quantum “complementarity” must be factored into our knowledge of the state of these two “q-on” systems.
Everyday language is ill suited to describe quantum complementarity, in part because everyday experience does not encounter it.
[2] I’m not sure whether the best characterization is that the photons are in the same (unified) state or share the “same state” or … that the photons are in one entangled quantum state or …
And this 2022 comment notes:
Quantum entanglement is when two particles link together in a certain way no matter how far apart they are in space. Their [quantum] state remains the same [i.e., correlated].
[3] I assume that the proper characterization of the entangled photons entails a single wave function rather than two identical wave functions (for identical localized excitations). The photons are distinct but act like – are mathematically treated as – a single “object.”
For example, something like:
Ψ = 𝜓1 (𝑋1) 𝜓2(𝑋2) when not entangled
Ψ(𝑋1, 𝑋2, 𝑡 ) when entangled (wave function is not factorizable)
where 𝑋1 and 𝑋2 constitute the coordinates of the two particles and for time t.
Also as noted in this StackExchange question:
• Physics Stack Exchange > “If two particles are entangled and you collapse the wave function of one of the particles, does the other particle’s collapse as well?” (March 9, 2017)
[Answer] Each photon does not have its own wave function. They are entangled. By definition, there is only one wave function between them. One function describes both particles simultaneously. If you do something to one particle that alters the wave function, then that’s it; the wave function is altered.
This 2017 comment lists ways to create quantum entanglement:
- Entanglement from birth
- Second-generation entanglement
- Entanglement by accident
- Entanglement by interaction
Spontaneous parametric down-conversion (SPDC) often is described as “splitting” photon beams into pairs of photons which are phase-matched (coupled) in the frequency domain and have correlated polarizations. (Wiki notes that “the conversion efficiency of SPDC is typically very low.”)
And this 2020 comment references research about creating single photons on demand, as well as transferring entanglement from one atom to another.
Wiki:
It is also possible to create entanglement between quantum systems that never directly interacted, through the use of entanglement swapping. Two independently prepared, identical particles may also be entangled if their wave functions merely spatially overlap, at least partially
The electron shells of multi-electron atoms always consist of entangled electrons. The correct ionization energy can be calculated only by consideration of electron entanglement.
References
The Caltech Weekly
• Caltech > Science Exchange > “What Is Entanglement and Why Is It Important?“
Like other aspects of quantum science, the phenomenon of entanglement reveals itself at very tiny, subatomic scales. When two particles, such as a pair of photons or electrons, become entangled, they remain connected even when separated by vast distances. In the same way that a ballet or tango emerges from individual dancers, entanglement arises from the connection between particles. It is what scientists call an emergent property.
The Caltech Weekly article also references a YouTube video from October 2021.
• YouTube > Caltech Science Exchange > “How Do Scientists Explain Quantum Entanglement?” (Oct 11, 2021)
(video description) In this video, four Caltech faculty members explain quantum entanglement, each sharing metaphors and concepts that help describe this mind-bending phenomenon.
Entanglement is at the heart of quantum physics and future quantum technologies. Like other quantum phenomena, entanglement reveals itself at tiny, subatomic scales. When two particles, such as a pair of photons or electrons, become entangled, they can remain connected even when separated by vast distances. In the same way that a ballet or tango emerges from individual dancers, entanglement arises from the connection between particles. It is what scientists call an emergent property.
Featured:
Rana Adhikari, professor of physics
Xie Chen, professor of theoretical physics
Manuel Endres, professor of physics and Rosenberg Scholar
John Preskill, Richard P. Feynman Professor of Theoretical Physics, Allen V. C. Davis and Lenabelle Davis Leadership Chair, and director of the Institute for Quantum Information and Matter
(from transcript)
entanglement is this weird phenomena.
the most fundamental form of correlations in the world.
they’re an object that started off in the same little pod or atom or whatever it was and now they’ve burst apart. [as an example] the two photons are only related to each other and they’re not disturbed by anything else in the universe yet, and so they are completely entangled, meaning you can treat them as a single object – you have to treat them like a single object.
quantum mechanical particles somehow still keep the memory of the [their] origin … where their states are correlated with each other; so that even when they are separated very far away, their states are still correlated.
[as an example] if something [one member of an entangled pair] points in a specific way, it [the other member of that pair] has to point in another way; or, it has to point exactly the same way. so, we call this a correlation, and entanglement is correlations on a quantum level, and the type of correlations you can get is fundamentally stronger than if you just have classical objects.
so it’s like that you have a twin and they’re born together and they look the same. they have a lot of attributes that are related to each other. but then later in life they might be separated far away, but then they still keep this relation throughout the years. and then they have a special way of communicating maybe with each other.
[start Preskill] imagine a book which contains many pages … if it’s an ordinary book, it’s written in bits. there’s writing on every page, you can read the pages one at a time; and every time you read another page, you learn more about the content of the book.
now suppose this book though is a quantum book. if that book is very highly entangled – if the pages are entangled with one another – there’s lots of information in the book; but it’s not stored in the individual pages – it’s stored almost entirely in the correlations [relationships] among the pages. and if you want to read the book you have to collectively observe many pages at once [grok the gestalt].
so that’s the way i look at it – the essence of quantum entanglement – that you can have a quantum system which stores a lot of information; but when you look at the parts of the system one at a time, you can’t see the information at all [because it’s an emergent relational group property]. [end Preskill]
it’s a very um precious thing. it’s very very hard to do because it’s very uh sensitive. so, you have two quantum particles in your lab, and you try to entangle them and something else flies by, even just close by, it’s going to be gone. it’s like it doesn’t like, you know, it doesn’t like companies [congregate] entanglement. it wants just to be by itself.
[practically speaking] the more things that we can entangle, and the more things that we can keep from getting disturbed by the rest of the world … and every year as we get better and better and better, we’re starting to do things which are almost you would have considered [as] magic just 50 years ago.
Notes from 2021
Elsewhere I’ve noted discussions of quantum entanglement, books and articles, and even videos on the subject. It’s a big deal.
Entanglement is real. No dispute about that. Technology is being developed based on entanglement. The implications for classical and quantum mechanics, however, is ongoing.
But why is it such a big deal? How explain that? With levels of understanding. And how can you prove some things are entangled?
I’ve pondered the analogies and both the logic and equations of John Stewart Bell’s theorem. My grasp of all that varies.
In our everyday world, we’ve expect there to be some type of mechanism. Stuff that’s interconnected somehow. Where connections can be defined. But we’re exploring a scale dominated by indeterminacy.
As a result, presentations focus on examples of probability. Like coin flips.
And experiments designed to test the quantum theory on the concept are complex.
Note: Typically involving statistical correlation between zillions of particles (like photons or electrons).
And most of the time, analogies are used to explain what’s being described. A lot of talk about “Alice & Bob.”
And that’s why this video below caught my attention. Especially when Philip Ball said, “Personally, I’ve never seen an explanation of John Bell‘s experiment that is at all easy to follow.” But then again, for many, even this may be like watching an entertaining magician.
*Bell’s theorem proves that quantum physics is incompatible with local hidden variable theories. It was introduced by physicist John Stewart Bell in a 1964 paper titled “On the Einstein Podolsky Rosen Paradox”, referring to a 1935 thought experiment that Albert Einstein, Boris Podolsky and Nathan Rosen used to argue that quantum physics is an “incomplete” theory.
It’s all about correlation. The Bell inequality.
*Bell then showed that quantum physics predicts correlations that violate this inequality. Consequently, the only way that hidden variables could explain the predictions of quantum physics is if they are “nonlocal”, somehow associated with both halves of the pair and able to carry influences instantly between them no matter how widely the two halves are separated. As Bell wrote later, “If [a hidden-variable theory] is local it will not agree with quantum mechanics, and if it agrees with quantum mechanics it will not be local.”
• Aeon digital magazine > Video > “Quantum entanglement is tough to dumb down, but this analogy can help detangle it” – a 20′ presentation by science writer Philip Ball (author of the 2018 book Beyond Weird: Why everything you thought you knew about quantum physics is different) on April 10, 2020 – by The Royal Institution (producer Anand Jagatia).
Description
The term ‘quantum entanglement’ refers to quantum particles being interdependent even when separated, to put it in exceedingly simple terms.
Because this behaviour was so at odds with his understanding of the laws of physics, Albert Einstein called the phenomenon ‘spooky action at a distance’.
And because it is so hard to square with our own lived experience, it is often used as one of the foremost examples of ‘quantum weirdness’.
In this expansion on a previous Royal Institution presentation, the UK science writer Philip Ball details a metaphor devised in the 1990s by Sandu Popescu, professor of physics at the University of Bristol, and Daniel Rohrlich, a physics researcher and lecturer at Ben-Gurion University of the Negev, to help bring our current best understanding of quantum entanglement into focus.
In doing so, Ball also provides an enlightening window into physicists’ evolving understanding of the quantum world throughout the 20th century.
Other references
• Space.com > “Quantum entanglement: A simple explanation” by Jesse Emspak, contributions from Kimberly Hickok (published March 15, 2022) – Quantum entanglement is one seriously long-distance relationship.
Quantum entanglement is a bizarre, counterintuitive phenomenon that explains how two subatomic particles can be intimately linked to each other even if separated by billions of light-years of space. Despite their vast separation, a change induced in one will affect the other.
• Washington Post > “Quantum entanglement, science’s ‘spookiest’ phenomenon, achieved in space” by Sarah Kaplan (June 15, 2017)
Luckily for quantum physicists, you don’t always need to explain a phenomenon in order to use it. Ancient humans didn’t know about friction before inventing the wheel; doctors in medieval China didn’t know about antibodies when they began inoculating people against smallpox 600 years ago. Not knowing what’s behind quantum entanglement didn’t stop Jian-Wei Pan, a physicist at the University of Science and Technology of China in Shanghai, from rocketing it into space.
Related posts
• How to create entangled photon pairs
This podcast with Sean Carroll re the quest for quantum gravity (cf. his latest book) includes an answer to what is entanglement.
• Quanta Magazine > “Where Do Space, Time and Gravity Come From?” by Steven Strogatz (May 4, 2022) – my note from podcast transcript.
Entanglement – “It’s not a rare, special thing.”
Without mentioning wave functions, here’s IEEE’s well-crafted take on the topic, which includes:
• IEEE > Spectrum > “What Is Quantum Entanglement?” by Dan Garist (8 June 2022) – Let’s talk about socks.
The article uses tables to illustrate Bell’s inequality.
Notes
[1] Nonlocality as real vs. illusionary.
[2] In other words, as noted above (in my post): “the quantum state of each particle of the group cannot be described independently of the state of the others.”
How is entanglement verified? In this case, by “evaluating more than one million measurements.”
As a useful historical recap and description of a new “big science” experiment using superconducting circuits – a loophole-free Bell test “showing that quantum mechanical objects that are far apart can be much more strongly correlated with each other than is possible in conventional [classical] systems.”
• Phys.org > “Entangled quantum circuits further disprove Einstein’s concept of local causality” by Felix Würsten, ETH Zurich (May 10, 2023)
How is entanglement verified between elementary (so-called) particles? Let alone those with a mean lifetime of 5×10^−25 second (less than a yoctosecond)?
• Earth.com > News > “‘Spooky action at a distance’ confirmed to persist between top quarks” by Eric Ralls (6-15-2024) – An overview of full study published recently in the CMS Physics Analysis Survey (13 June 2024).
This article describes a research milestone in creating on-demand entangled photon pairs using semiconductor quantum (dot) emitters (vs. crystal-based spontaneous parametric down-conversion). The writer characterizes the pair as “linked.”
• Phys.org > “New light source emits bright, entangled photons for quantum communication” by TranSpread (August 5, 2024)
Related articles
• Nature > “Robust, high brightness, degenerate entangled photon source at room temperature” by M. V. Jabir & G. K. Samanta (October 3, 2017)
• Lund University Publications > “A high brightness source of entangled photons” [pdf] (Spring 2010)
1 Introduction
1.1 History
1.2 Photonic entanglement
1.3 Different forms of entanglement
1.3.1 Momentum
1.3.2 Energy-Time
1.3.3 Polarization
1.4 Measuring entanglement
1.4.1 Coincidence fringe visibility
1.4.2 Bell’s inequality
Related questions
• How are entangled photons measured?
• How do you know if two particles are entangled?