[“Quest” series]
This is a topic which I’ve followed for decades. A holy grail of physics: room temperature superconductivity. The physics of Cooper pairs: what makes two electrons pair up when their charge actually makes them repel each other?
SciTechDaily > “Breakthrough in Understanding the Physics of High-Temperature Superconductivity” by Helmholtz-Zentrum Dresden-Rossendorf (May 12, 2020).
How do electrons form pairs in high-temperature superconductors such as cuprates?
In superconductivity, electrons combine to create “Cooper pairs,” which enables them to move through the material in pairs without any interaction with their environment. But what makes two electrons pair up when their charge actually makes them repel each other?
For conventional superconductors, there is a physical explanation: … One electron distorts the crystal lattice, which then attracts the second electron. For cuprates, however, it has so far been unclear which mechanism acts in the place of lattice vibrations.
At this point “Higgs oscillations” enter the stage: In high-energy physics, they explain why elementary particles have mass. But they also occur in superconductors, where they can be excited by strong laser pulses. They represent the oscillations of the order parameter – the measure of a material’s superconductive state, in other words, the density of the Cooper pairs.
So, “Higgs oscillations” = oscillations in the density of the Cooper pairs? Such oscillation can be induced via cyclic high-energy laser pulses. Like using your legs to continuously maintain a swing, eh.
[Image caption] By applying a strong terahertz pulse (frequency ω), they stimulated and continuously maintained Higgs oscillations in the material (2ω). Driving the system resonant to the Eigenfrequency of the Higgs oscillations in turn leads to the generation of characteristic terahertz light with tripled frequency (3ω). Credit: HZDR / Juniks
A landmark in condensed matter physics …
• Quanta Magazine > “Room-Temperature Superconductivity Achieved for the First Time” by Charlie Wood (October 14, 2020) – Physicists have reached a long-sought goal. The catch is that their room-temperature superconductor requires crushing pressures to keep from falling apart [maintain a metallic lattice].
[September 2022 update]
As noted below, the study profiled in Wood’s article re superconductivity research was retracted by the journal Nature. As Neil deGrasse Tyson says, that’s par for the bleeding edge of scientific research.
• Science.org > “‘Something is seriously wrong’: Room-temperature superconductivity study retracted” by Eris Hand (Sep 26, 2022) – After doubts grew, blockbuster Nature paper is withdrawn over objections of study team
More research on terahertz quantum cascade lasers …
• Phys.org > “Researchers develop a high-power, portable terahertz laser” by Michaela Jarvis, Massachusetts Institute of Technology (Nov 2, 2020)
Terms
Phonon
Quantum well structure
The quest continues for room temperature superconductivity, with yttrium hydride (YH6) requiring less extreme pressure than the reigning leader. [See prior Oct 18, 2020 comment.] A new puzzle as to why “the critical magnetic field observed in the experiment is 2 to 2.5 times greater as compared to theoretical predictions.” A goal to attain superconductivity at lower extreme pressures.
• EurekAlert (American Association for the Advancement of Science) > “Scientists have synthesized a new high-temperature superconductor” by SKolkovo Institute of Science and Technology (Skoltech) (March 10, 2021)
Research on superconductivity in twisted bilayer graphene continues. All hail, Cooper pairs!
• Phys.org > “Research finds surprising electron interaction in ‘magic-angle’ graphene” by Kevin Stacey, Brown University (March 19, 2021)
Visualization
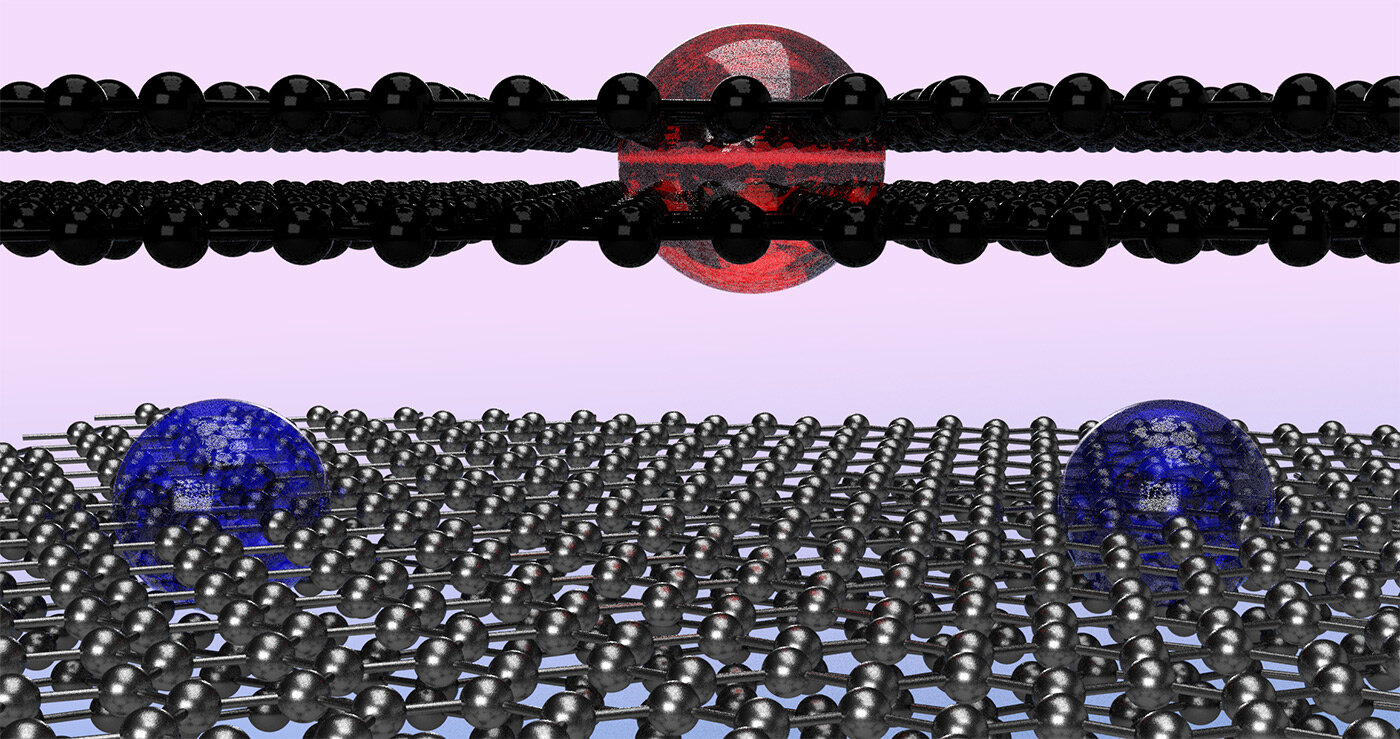
[Caption] Researchers have discovered a way to manipulate the repulsive force between electrons in “magic-angle” graphene, which provides new insight into how this material is able to conduct electricity with zero resistance. Credit: Li lab / Brown University
Continued research on magic graphene … using “a scanning tunneling microscope (STM) to view the infinitesimally small and complex world of electrons.”
• Phys.org > “Unmasking the magic of superconductivity in twisted graphene” by Princeton University (October 20, 2021)
A breakthrough discovery in understanding high-temperature superconductors models breaking a previously “hidden” particle-hole symmetry.
• Phys.org > “Physicists elucidate connection between symmetry and Mott physics” (the physics underlying high-temperature superconductors) by University of Illinois at Urbana-Champaign (March 22, 2022)
Terms
• Bardeen-Cooper-Schrieffer (BCS) theory
• Fermi liquid theory
• Hubbard model
• Hatsugai-Kohmoto model
More research on twisted graphene – understanding why not all twists achieve superconductivity.
• Phys.org > “In a sea of magic angles, ‘twistons’ keep electrons flowing through three layers of graphene” by Ellen Neff, Columbia University Quantum Initiative (April 8, 2022)
Caption: In order to model how electrons pair up in a superconducting material, MIT physicists captured 1000’s of images of particles pairing up in clouds of a very dilute gas of about 1,000 potassium-40 atoms. In this data visualization, the red and blue balls are spin-up and spin-down fermions, and some are paired together. The white sites are doubly occupied sites. Credit: Thomas Hartke, MIT.
Here’s an interesting article on visualizing electron pairing with analogous systems of fermion atoms – to better understand superconductivity. After a years-long team effort by members of MIT’s Department of Physics, the MIT-Harvard Center for Ultracold Atoms, and the Research Laboratory of Electronics.
Terms: Hubbard model, optical lattice
• Phys.or > “Physicists generate first snapshots of fermion pairs, shed light on how electrons form superconducting pairs” by Jennifer Chu, MIT (July 6, 2023)