[“Quantum foundations” series] [Updated December 2019]
Introduction to this topic
If Murray Gell-Mann was right that Niels Bohr brainwashed a generation of physicists to accept the Copenhagen Interpretation, either his influence has waned or he didn’t do a very good job in the first place.
For in an informal poll conducted at an international meeting in 2011 on ‘quantum physics and the nature of reality’, fewer than half of the attendees professed an allegiance to Bohr’s position. True, his was still the most popular interpretation by a considerable margin. But it can hardly claim to represent a consensus.
Sixteen years previously, another show of hands had been taken by the MIT physicist Max Tegmark at a similar meeting in Maryland. The Copenhagen Interpretation triumphed on that occasion too, albeit also without a majority. But Tegmark was delighted to note that in second place was his own favoured view of quantum mechanics: the Many Worlds Interpretation (MWI).*1 – Ball, Philip. Beyond Weird (p. 288). University of Chicago Press. Kindle Edition. [*1 It’s been suggested, however, that what these polls really tell you is who organized the meeting. – Ibid (p. 360)]
Review
Theoretical physicist Sean Carroll recently published a new book. I’ve read some of his books. His blog, podcast. Articles. He has a robust online media presence. And is skilled at promoting his new book (or has a skilled publicist). So, I’ve read several articles on different sites about his new book, as well as downloaded a Kindle sample of the book.
Here’re a few examples of articles promoting the new book, Something Deeply Hidden: Quantum Worlds and the Emergence of Spacetime.
• PBS NewsHour: YouTube video “Sean Carroll: Universe a ‘tiny sliver’ of all there is” (published on Sep 15, 2019)
• NPR: In ‘Something Deeply Hidden,’ Sean Carroll Argues There Are Infinite Copies of You (September 13, 2019) by Adam Frank, astrophysics professor at the University of Rochester
• Whatever: The Big Idea: Sean Carroll (September 12, 2019) by John Scalzi, author of the “Old Man’s War” series of novels
• Wired: Sean Carroll Thinks We All Exist on Multiple Worlds (September 10, 2019) by Sophia Chen
• New York Times: Even Physicists Don’t Understand Quantum Mechanics
Worse, they don’t seem to want to understand it (September 7, 2019) by Sean Carroll, theoretical physicist at the California Institute of Technology
After almost a century of pretending that understanding quantum mechanics isn’t a crucial task for physicists, we need to take this challenge seriously.
I found NPR’s book review particularly interesting. That article recaps around a 100 year old debate about quantum mechanics and realism, a debate harkening back to its original seminal figures and regaining new life in the last few years after decades of what has been characterized as “shut up and calculate” successes.
There are a variety of reasons that this debate about quantum foundations – foundational questions related to quantum mechanics (QM) and quantum theory – has renewed vigor. Often cited are the rarified mathematics of current theories (particularly those pushing beyond the Standard Model), questions about the future of particle physics (viability of further discoveries by the LHC and investment in even greater colliders), and new perspectives by some modern well-known physicists.
The NPR review notes that no matter what your QM interpretation or perspective is (or take on the many popular tropes about what QM says), Carroll’s emphasis is that foundational questions be returned from the backwaters of legitimate research – whether you agree with his advocacy of the Everettian many-worlds interpretation of QM.
Carroll’s Everettian many-worlds perspective is included in his July 10, 2018, podcast “Episode 2: Carlo Rovelli on Quantum Mechanics, Spacetime, and Reality” – an interview with Carlo Rovelli. [1] Here’s an excerpt.
Carroll: But it’s not so far from mine in that part in the sense that I am an Everettian, a believer in the many worlds interpretation, at least I think that’s my favorite. I’m happy to change my mind some day down the road, but Everett himself, when he invented it in the 1950s, when he published his paper, he called it the relative state interpretation. And the point that he was trying to emphasize was that what you see is only relative to the thing seeing it. This interaction creates an impression of the measurement outcome, that is intrinsically part of the relationship between the thing that is doing the measuring and the thing that is being observed. So I think that there’s some similarity of words there.
Rovelli: Some similarities. I shouldn’t talk for you, but let me try it for one second. I think what you would add to what I say, is that you want to take a realist position where beyond that there is nevertheless a deterministic non-relational overall quantum state of the system, that we may take as the proper description of reality.
Carroll: I would like to say that, yeah …
Rovelli: Would that be so?
Carroll: Yeah, I would. I’m a realist. I can’t even imagine what it would be like to be a scientist and not be a realist about reality. [laughter] And yet I know …
Rovelli: Right, so I would like to be a realist, but less radically so. …
And so I find that if we want to be a realist about the wave function, we have … Actually, let me step back for a moment. I think, given the strangeness of quantum mechanics, any way we think it is, comes with a price. So we have options. We have a number of options, and we have been talking about two options, okay, the sort of many world interpretation, in which you have this wave function of everything, which come with a price, or the sort of strictly relational view which I described, which also come with a price. And there are others and they have their own price.
Carroll: Right, that’s right.
Rovelli: And many discussions about quantum mechanics are, “Well, which price are we ready to pay?” And the price of the relational interpretation I described is this weakening of realism. So to say, what the state of the system … Well, I don’t know, it doesn’t matter, it doesn’t have a state, it only has a state with respect to A, and it is the same with respect B, and it is the same with respect to C, and there is no solid objective, overall picture. The price of the many world interpretation, is that I have to accept …
Carroll: Many worlds.
Rovelli: In some sense all these many worlds.
Carroll: Yes, absolutely, it’s a big price, it’s a big price.
Rovelli: So it comes with an enormous ontology, a very heavy ontology, and which price do we wanna pay. In one way or the other, it contradicts our previous way of thinking of reality.
Carroll: Absolutely, and I … So I couldn’t agree more about the idea that there is a price to be paid. I could disagree a little bit about the heaviness of the ontology that is associated with many worlds, but I think that this is a good lesson, because all we need for today’s conversation is the clear fact that we have at the same time, a way to do quantum mechanics, we use quantum mechanics as physicists every day, it’s been spectacularly verified experimentally. We know how to use it, we have a recipe, we have a black box.
Rovelli: Yeah, and it works, it works fantastically well.
Carroll: And we don’t have anything close to an agreement on what it is, what the fundamentals of it really say. So that will be a wonderful thing to figure out once and for all, but maybe we don’t need to do that for the question of quantizing gravity. So just to put the puzzle, the task, in perspective, since we grew up, we human beings, as classical people, classical mechanics was invented first. It is very intuitive to us, even if pendulums and inclined planes tortured us as undergraduates, we kind of get it in a fundamental, visceral way. So we always start by thinking about systems from this classical point of view and then we quantize them, we elevate the classical description to some quantum mechanical theory. Now, presumably nature or God doesn’t work that way.
Okay, what’s my personal take on this topic? Well, I agree that quantum foundations is important. Studying philosophy (including philosophy of science) has shaped my perspective. Generally, I side more with Rovelli than Carroll on realism and QM.
Carroll is well grounded in the mathematical framework of QM, and my grasp of that is all too limited. I sometimes think that his conviction (no matter how provisional) is based on mathematics for which any explanation to “mere mortals” is problematical; so, metaphorical language misses the core of the argument.
[Carroll] And we look at and say yes, I see the worlds, there they are. I see them in my math. I don’t see them in my telescopes or microscopes.
The many-worlds interpretation focuses on the so-called measurement problem (wave function collapse), and there are many tropes which blend the terms measurement, observation, and even decision (by the PBS NewsHour) – as splitting other worlds. Carroll is careful in discussing this point, repeatedly stating that it’s an open question as to what “measurement” means (what counts as an “observation” or whether consciousness is required or not, for example). Yet, I thought that advances in understanding decoherence had diffused the problem. As Philip Ball said, “The universe is always looking.” [3]
And indeed the PBS NewsHours’ characterization that QM claims that “two objects can occupy the exact same space at the same time” is misleading. Their use of the words “object” and “exact” miss even a visualization of radio waves (EM photons) overlapping or interfering: positional and spatial concepts of waves – wavelength and superposition. Discussing wave functions is even more arcane.
[Carroll] But what is the wave function? Is it a complete and comprehensive representation of the world? Or do we need additional physical quantities to fully capture reality, as Albert Einstein and others suspected? Or does the wave function have no direct connection with reality at all, merely characterizing our personal ignorance about what we will eventually measure in our experiments?
So, Carroll talks about the measurment problem. And Rovelli’s Relational quantum mechanics replaces measurement with interaction as the way to remain a “realist about the wave function.” That is, a “property becomes concrete when two systems interact.”
You replace measurement with interaction. But to make the machine work, you have to assume that when two systems interact, the property of one system becomes actual, with respect to the other system, and not with respect to everything else. And that point is really shocking.
There are a lot of quantities in physics which are relational, we know that they are. The velocity of an object is not a property of the object. There’s no sense in which the velocity of an object is a property of the object … The velocity of an object is a property of an object with respect to another object.
So I can say that the Earth is set to velocity with respect to the Sun, but the Sun is not measuring, it’s not going out with meters and kilometers. So we use this language, measuring, and we interpret this language to say that some properties are relational. I want to be a realist about relational properties. And I want to think of reality in a realistic way … to our direct experience of reality [observables]. I see the photons coming in, I see the table being the table …
But in his book, Carroll defines his Courageous Formulation of an Austere Quantum Mechanics this way:
This minimalist approach has two aspects. First, we take the wave function seriously as a direct representation of reality, not just a bookkeeping device to help us organize our knowledge. We treat it as ontological, not epistemic. That’s the most austere strategy we can imagine adopting, since anything else would posit additional structure over and above the wave function.
Personally I am not sure about the reality of the wave function – as ontic vs. epistemic.
Wiki: “If the wave function is physically real, in some sense and to some extent, then the collapse of the wave function is also seen as a real process, to the same extent.”
Fermilab’s Don Lincoln takes on this topic in his November 18, 2019, YouTube video “What is quantum mechanics really all about?” (below). In particular, regarding the reality of the wave function and its so-called collapse.
Additional videos from Carroll’s book tour
• Chicago Humanities Festival > Sean Carroll: Many Worlds (published November 14, 2019 on YouTube)
Additional critiques of Carroll’s book
• Forbes > “Many Worlds, But Too Much Metaphor” by Chad Orzel, Contributor (Sep 17, 2019)
Orzel discusses the notion of a wavefunction of the universe (“in an indescribably complex superposition”), localized superposition, probability, measurement, and decoherence.
That process of interaction with the changing state of an unknown environment gets the name “decoherence,” and it’s what enables the bookkeeping trick that lets us split off pieces of the wavefunction and consider them in isolation. If the piece you’re interested in is big enough and interacts with the environment strongly enough, there’s no hope of doing the interference measurement that would show it’s in a superposition state. If you can’t do a measurement that would show the existence of the other piece(s) of the superposition, you can safely treat it as being in a single definite state.
Orzel sees the math much as Carroll does. But he concludes that the typical language in which the Many-Worlds Interpretation (MWI) is cast takes us down a counterproductive rabbit hole (much like the “rubber sheet” analogy for spacetime curvature in General Relativity). As mere mortals, we tend to become enchanted with a vivid metaphor – too literally – because the mathematics is so impenetrable.
As Philip Ball says in his book: “The Many Worlds Interpretation denies language, but gets away with it because language has a notorious capacity to express things that appear to have meaning yet do not. – Ball, Philip. Beyond Weird (p. 360). University of Chicago Press. Kindle Edition.
Orzel suggests an alternative perspective on “an otherwise incomprehensibly vast wavefunction.”
I actually like the start of Carroll’s presentation quite a bit, where he casts MWI as “Austere Quantum Mechanics,” with the only postulates being that the universe is described by a wavefunction, and that the wavefunction evolves according to the Schrödinger equation.
The problem is that after that austere beginning, Carroll dives back into the somewhat baroque metaphor that’s grown up around the simple initial idea, talking at great length about branches of the wavefunction that contain copies of everything in the universe that differ only in the results of particular measurements. This language is really an additional interpretive superstructure on top of the actual austerity of MWI, an extended metaphor for the experience of observers within the theory. It’s also where everything goes wrong, from the standpoint of communication.
Talking about “parallel worlds” or even “branches of the wavefunction” as real separate things invites a whole bunch of questions that are really about the metaphor, not the theory, and thus ultimately unproductive.
Thinking about MWI in this way – as a bookkeeping trick to simplify an otherwise incomprehensibly vast wavefunction – clears up most of the typical objections that arise from taking the “separate worlds” metaphor too literally. There’s no “Occam’s Razor” problem because there’s only one wavefunction obeying one set of rules. There’s no issue with “creating copies” of everything, because there are no copies: there’s one universe, with one set of components described by one wavefunction. It’s not even a problem that the criteria for “splitting” are kind of nebulous, because it’s clear that it’s a fundamentally arbitrary process – the choice of which pieces to isolate and discuss is purely a matter of bookkeeping convention for the convenience of puny human physicists.
So, that’s my argument for why the way we talk about the Everettian interpretation of quantum mechanics sucks, and should be revisited. Please note that I’m not saying that Sean Carroll or any of the other super-smart people who spend time and energy thinking about and working with MWI are Doing It Wrong in terms of the physics – mathematically, thinking of the different pieces we can carve out of the giant wavefunction of the universe as separate branches works perfectly well. That’s how you keep the books. All I’m arguing is that, on a conceptual level and in terms of the language used to communicate to non-experts, we should do a better job of making clear that it is just bookkeeping.
Rather than “Many-Worlds Interpretation,” I’d go with “Metaphorical Worlds Interpretation,” to reflect the fact that all the different ways of cutting up the wavefunction into sub-parts are fundamentally a matter of convenience, a choice to talk about pieces of the wavefunction as if they were separate, because the whole is too vast to comprehend.
• Quanta Magazine > “Why the Many-Worlds Interpretation Has Many Problems” by Philip Ball (October 18, 2018) > The idea that the universe splits into multiple realities with every measurement has become an increasingly popular proposed solution to the mysteries of quantum mechanics. But this “many-worlds interpretation” is incoherent, Philip Ball argues in this adapted excerpt from his new book Beyond Weird.
Ball’s article recaps the history of the Many-Worlds Interpretation (vs. multiverse hypothesis), with references to Bohr, Everett, DeWitt, Deutsch, Greene, Vaidman, Wallace … And how to characterize the breakdown of superpositions – as separations, splits, parallels, alternative outcomes, bifurcations, copies, etc. And how the theory of decoherence “helped to revitalize the MWI by supplying a clear rationale for what previously seemed a rather vague contingency.”
But he then notes that MWI’s theoretical austerity (“parsimony of assumptions”) does not banish conceptual and metaphysical problems.
My own view is that the problems with the MWI are overwhelming — not because they show it must be wrong, but because they render it incoherent. It simply cannot be articulated meaningfully.
As Carroll does in his book tour (as in the Chicago Humanities Festival video from November 2019), Ball distinguishes between weak objections to MWI and strong ones. The better objections include:
• The insouciance with which MWI is cast – the casual egalitarianism of each of an infinty of infinte atomic quantum “measurements” (and the weighting of all those countless “Butterfly effects” on everyday macro objects and creatures).
• The notion of self and doppelgänger tropes and consciousness: “the level of the discourse about our alleged replica selves is often shockingly shallow.”
• The interpretation of probabilities: “If all outcomes occur with 100-percent probability, where does that leave the probabilistic character of quantum mechanics?”
He concludes that: “We are not just suspended in language; we have denied language any agency. The MWI — if taken seriously — is unthinkable.”
But (as Carroll might agree), Ball notes that MWI “should be valued for forcing us to confront some tough philosophical questions.”
Additional note
The chapter “There is no other ‘quantum’ you” in Ball’s book examines the Many-Worlds Interpretation (MWI) and the Everettian vision of the entire universe as an immeasurable incomprehensible wavefunction.
As [the ‘universal wavefunction’] evolves, some of these superpositions break down, making certain realities distinct and isolated from one another. In this sense, … we should speak of the unravelling of two realities that were previously just possible futures of a single reality.
And he notes the foundational question regarding reality of mathematical constructs (akin to Plato’s theory of Forms):
• The reification of Hilbert space: “Hilbert space – the mathematical construct that contains all the possible solutions of the variables in the Schrödinger equation … is a construct – a piece of math, not a place.”
Elsewhere is his book, he writes: “… the notion of a universal wavefunction is popular with cosmologists, for the perfectly valid reason that in the earliest moments of the Big Bang the entire universe was smaller than an atom and surely needs to be considered, in that moment, a quantum-mechanical entity.”
Related posts
Quantum trajectory theory?
Feynman’s legacy – quantum originality
The future of (particle) physics?
Related pages
Online video: Perimeter Institute Lee Smolin Public Lecture “Einstein’s Unfinished Revolution” (published on Apr 18, 2019)
Notes
[1] Other podcasts by Sean Carroll:
- Episode 63 Solo: Finding Gravity Within Quantum Mechanics, September 9, 2019
- Episode 59 Adam Becker on the Curious History of Quantum Mechanics, August 12, 2019
- Episode 55 A Conversation with Rob Reid on Quantum Mechanics and Many Worlds, July 15, 2019
- Episode 36 David Albert on Quantum Measurement and the Problems with Many-Worlds, March 4, 2019
- Episode 31 Brian Greene on the Multiverse, Inflation, and the String Theory Landscape, January 28, 2019
[2] From Carroll’s Research overview page:
These days my physics research is a bit different, focusing on two big themes. The first theme is the foundations of quantum mechanics, especially connections to cosmology and emergent spacetime. I’m a proponent of the Everett (“many-worlds”) approach to quantum mechanics. It’s a great theory, but raises a number of questions to which the answers are not yet clear, including the origin of probability and how the furniture of our semi-classical world (space, time, fields) emerges from an underlying wave function in Hilbert space.
[3] Wiki: Quantum decoherence
However, decoherence by itself may not give a complete solution of the measurement problem, since all components of the wave function still exist in a global superposition, which is explicitly acknowledged in the many-worlds interpretation. All decoherence explains, in this view, is why these coherences are no longer available for inspection by local observers. To present a solution to the measurement problem in most interpretations of quantum mechanics, decoherence must be supplied with some nontrivial interpretational considerations (as for example Wojciech Zurek tends to do in his existential interpretation). However, according to Everett and DeWitt, the many-worlds interpretation can be derived from the formalism alone, in which case no extra interpretational layer is required.
http://www.toutestquantique.fr for other animations and explanations about quantum physics
Realisation Data-Burger, scientific advisor: J. Bobroff, with the support of : Univ. Paris Sud, SFP, Triangle de la Physique, PALM, Sciences à l’Ecole, ICAM-I2CAM
Copyright Bobroff 2012
[5] Quantum superposition of states and decoherence
See other animations at http://www.QuantumMadeSimple.com
Animations produced by the research groupe www.PhysicsReimagined.com with support of labex PALM.
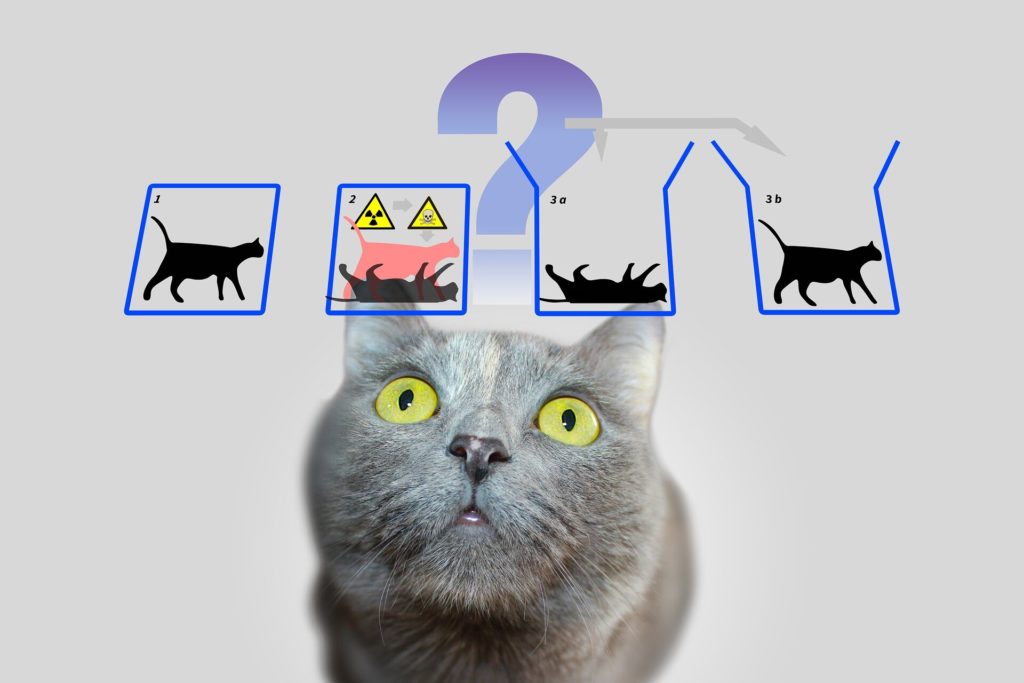
phys.org > “Deconstructing Schrödinger’s cat” by Springer (February 14, 2020)
The idea of linking quantum collapse to gravity has already been proposed by the great English physicist and philosopher Roger Penrose, but he never developed his ideas into a complete theory. Laloë proposes a model that goes in the same direction, agrees with physical observations and may one day prove testable experimentally.
Scientific American > Quantum Physics May Be Even Spookier Than You Think – A new experiment hints at surprising hidden mechanics of quantum superpositions by Philip Ball (May 21, 2018)
So, that was in 2018. What happened?
A spin in the mix of art and science …
In the realm of fictional novels and literary worlds, this Open Culture article from 2014 discusses famous author Philip K. Dick‘s view of many worlds in his novels and personal experience: Philip K. Dick Theorizes The Matrix in 1977, Declares That We Live in “A Computer-Programmed Reality” (February 3rd, 2014). The article contains an edited video of a 1977 interview.
YouTube video
Title: Philip K Dick – SIMULATION THEORY
Published on Jul 11, 2017
Channel: Were We Lied To
As noted in Wiki:
Carroll mentioned that personal identity is an issue with the many-worlds interpretation.
On September 21, 2019, Sean Carroll added a post to his blog: “The Notorious Delayed-Choice Quantum Eraser.” The post is sort of an appendix to his new book. He discusses an alternative double-slit experiment (the experiment which Feynman characterized as the “heart of quantum mechanics”).
As Wiki notes, the delayed-choice quantum eraser experiment, first performed in 1999, investigates a paradox.
Detecting through which slit a photon goes – the which-way experiment – results in no interference pattern. “This which-way experiment illustrates the complementarity principle that photons can behave as either particles or waves, but not both at the same time.”
Wiki also notes the recent use of entangled photons: “in order to avoid any possible ambiguity concerning the quantum versus classical interpretation, most experimenters have opted to use nonclassical entangled-photon light sources to demonstrate quantum erasers with no classical analog.”
While the result of the delayed-choice quantum eraser is similar to that of the basic double-slit experiment, the significance is harder to understand. There’s more logic involved in decoding the data. The experiment uses entangled “signal” and “idler” photons. More photon paths – more beam splitters and detectors. And a coincidence counter which helps filter – “pull out” (recover) the interference (pattern) from – the photon mixture.
I liked Carroll’s blog post. His short story of the (thought) experiment is crisper than Wiki’s discussion (above). He uses entangled “traveling” and “recorder” photons. And quantum state Ψ notation.
The post helped me understand his many-worlds stance better. Connecting interference, bookended entanglement, and decoherence. (He says, “Entanglement of any sort kills interference.”)
It’s refreshing to see Carroll use some visuals (tables and diagrams) in chapter one of his book to characterize the differences between classical and quantum physics. Maybe a visualization of an identical simple system for each set of rules? – using modern measuring apparatuses in each case.
Certainly the so-called measurement problem is important. Even more interesting for me is the evolution of physics from (1) a mechanics of the time evolution of positions & velocities of macroscopic objects responding to “forces” to (2) that of positions & momentum of objects responding to energy dynamics to (3) that of energy wave functions which yield probabilistic measurements.
References
Wiki > Delayed-choice quantum eraser
Wiki > Complementarity (physics)
Wiki > Laplace’s demon
Wiki > Wave function collapse
And speaking of the double-slit experiment as witness to the “heart of quantum mechanics” (Feynman), here’s another Space.com article by Paul Sutter: “Is It a Wave or a Particle? It’s Both, Sort Of” (September 30, 2019). The article has some useful visualizations.
Sutter recaps the essential notions of a particle and a wave. A particle is a localized object which can “bounce” off other objects. A wave is not localized – it’s spread out. Waves wiggle and interfere with each other rather than bounce off each other. “Both waves and particles are described by very, very different sets of mathematical equations.”
Then Louis de Broglie proposed that matter particles have a wavelength. And later the double-slit experiment with an electron beam exhibited interference. [1]
In the deep mathematics of quantum mechanics, an electron (an electron’s wave function) is “a [delocalized] wave of probability representing all the possible places where a particle might be the next time we go looking for it” or “a cloud of where it might be” – something which can interfere with itself (as in the double-slit experiment).
Sutter jokes about being careful not to throw ourselves through narrow slits. But he doesn’t discuss the experiments which research how far we can go with the size of particles in double-slit experiments, as noted in my comment about 2000-atom molecules. That limit is being explored.
Why can’t we beam golf balls, baseballs, tennis balls, etc., and demonstrate interference?
The Wired article hints at the difficulty of maintaining the molecules in a coherent state. Tricky.
And if the wavelength is too small to measure:
Notes
[1] And the whole basis of modern transmission electron microscopes in fact depends on the waviness of electrons (so-called point particles) – that the wavelength of electrons is smaller than light.
Wiki >
Wiki > Matter wave
Wiki > Quantum coherence
And here’s perhaps the source of the image at the top of Sutter’s article (noted in my previous comment): LiveScience > “Image Captures Light’s Spooky Dual Nature for 1st Time” by Tia Ghose (March 3, 2015).
It’s a fascinating image indeed. I’d need to read commentary on the original journal article to understand LiveScience’s description of the experiment (below).
[Question: The photons changed speed?]
Reference
Wiki > Wave–particle duality
Last month, in another article promoting his new book, Sean Carroll discussed probability and how that concept is used in quantum mechanics.
Quanta Magazine > “Where Quantum Probability Comes From” by Sean Carroll, Contributing Columnist (September 9, 2019) > There are many different ways to think about probability. Quantum mechanics embodies them all.
References
Born rule
Bayesianism
And following up Carroll’s article on quantum mechanics and probability is this article on probability for mere mortals.
Towards Data Science > “Probability Theory 101 for Dummies like Me” by Sangeet Moy Das (Oct 13, 2019)
The article discusses these concepts:
Probability (as likelihood in tossing a coin, expressed as percents or fractions).
Probability (frequentist) – frequencies of outcomes in large samples or repeated trials over time.
Probability (subjective) – a measure of belief.
Random variable – a variable whose value is determined by chance (typically within some range of values).
Discrete random variable – a random varible whose values are specific and countable and not continuous, e.g., the outcome of a coin toss is either 0 (tails) or 1 (heads). Values are quantized.
Continuous random variable – values can vary continuously, so that probabilities can be related to the area under the curve of a probability density function.
Sample space – the collection of all possible outcomes.
Complement – the probability of the complement of A includes the sum of all probabilities in the sample space that is not A.
Mutually exclusive events (disjoint events).
Independent events.
Dependent events.
And the rules or axioms of probabiity (sometimes using a deck of cards and lastly a game of Roulette):
The range of probabilties and summation of those outcomes.
Arithmetic on probabiities.
The Law of Large Numbers.
The types of probability: marginal, joint (cf. Venn Diagram), conditional.
Bayes’ Theorem (for conditional probabilities).
And the article closes with a discussion of the difference between probability and statistics.
Frequentist vs. Bayesian View
Regarding Chad Orzel’s Forbes September 17, 2019, article noted above, here’s an excerpt from his earlier September 11, 2019, article:
Forbes > “What I Was Wrong About In Physics” by Chad Orzel Contributor (Sep 11, 2019)
But I’m not sure Carroll and Orzel are on the same page as to the reality of the wavefunction. Orzel’s statement:
appears contrary to what Carroll writes in his book on the matter regarding his Courageous Formulation of an Austere Quantum Mechanics:
SciTechDaily > “Pair of Civil Servants Rewrite Quantum Mechanics in Their Spare Time” by Aalto University (January 5, 2020).
Nature.com > Article | Open Access | Published: 27 December 2019 > “Quantum Mechanics can be understood through stochastic optimization on spacetimes” by Jussi Lindgren & Jukka Liukkonen in Scientific Reports volume 9, Article number: 19984 (2019).
SciTechDaily presents the background for the research paper but the paper itself is available elsewhere (as noted above).
Lindgren & Liukkonen’s research is connected to the exploration of quantum foundations (as in Sean Carroll’s latest book); and, in particular, the debate about the ontological status of the wave equation.
SciTechDaily summarizes the story of Lindgren & Liukkonen’s dissatisfaction with certain postulates of quantum mechanics: “… you had to accept they were true without it being shown why.” And how they “devised a new method for expressing the laws of quantum mechanics using stochastic methods, a type of mathematics that deals with random chance and probability.”
The paper itself defines a fuller context for the research.
Here’s their abstract:
The paper contains impenetrable mathematics beyond my ken, but the general framework is noteworthy.
Lindgren & Liukkonen grabbed my attention by noting the mathematical similarity of the Schrödinger equation to the ordinary diffusion equation, since I tend to explore quantum mechanics from a fluid dynamics perspective.
And they discuss in particular how imaginary time (as in complex algebra) arises in the mathematics.
Highlights
Notes
[1] See also arXiv.org > quant-ph > arXiv:1811.01107 – “Does the PBR Theorem Rule Out a Statistical Understanding of Quantum Mechanics?” by Anthony Rizzi (Submitted on 2 Nov 2018).
Terms
Action (physics)
Markov chain
Transition probability density
Sum over all paths
Relativistic invariance
Minkowski spacetime, Minkowski metric
Four-dimensional diffusion
Metric tensor
Planck scale
Hamiltonian, Lagrangian
Einstein summation
Stueckelberg covariant wave equation
Stochastic control scheme
Dirac equation
Telegrapher’s equation
Born rule
How big can an object be and still exhibit quantum behaviors?
Smithsonianmag.com > “A New Experiment Hopes to Solve Quantum Mechanics’ Biggest Mystery” by Ramin Skibba – Physicists will try to observe quantum properties of superposition—existing in two states at once—on a larger object than ever before (February 5, 2020).
Wiki:
Here’s a fascinating update on the double-slit experiment, noting its history and a recent innovative demonstration of interference for single atoms.
Physics World > “Double slits with single atoms” by Andrew Murray, professor of atomic physics in the Photon Science Institute, Department of Physics and Astronomy, University of Manchester, UK (February 18, 2020) – Thomas Young’s double-slit experiment is famous for demonstrating the principle of interference. Andrew Murray explains why it’s now possible to carry out an equivalent experiment using lasers that have excited individual rubidium atoms.
YouTube > Sabine Hossenfelder > “The Trouble with Many Worlds” (Sep 26, 2019) – “In today’s video I want to tell you why I am not a fan of the many worlds interpretation of quantum mechanics. … It’s that I think you do not gain anything from this reinterpretation.”
(from transcript)
Another take on the standing of key mathematical models in physics – whether representation is reality. With references to Eugene Wigner.
When a mathematical model works so well (as an effective theory), does that mean it reflects reality?
A model is by definition a simplification, eh? And works contextually. A practical (predictive) tool. What of bolder claims as to the nature of reality itself? So, Ptolemy-like craftsmanship or Plato-like revelation?
• Scientific American > Math > Opinion > “Is the Schrödinger Equation True?” by John Horgan [1] (January 7, 2021)
Notes
[1] (“About the author” blurb) John Horgan directs the Center for Science Writings at the Stevens Institute of Technology. His books include The End of Science, The End of War and Mind-Body Problems, available for free at mindbodyproblems.com. For many years, he wrote the immensely popular blog Cross Check for Scientific American.
Related posts
Quantum mechanics math basics – tasting the notation
Cosmological fact and fiction
Eigen what?
Here’s an article about preserving quantum state coherence in practical applications.
• Phys.org > “Researchers set record by preserving quantum states for more than 5 seconds” by Argonne National Laboratory (February 2, 2022)
So, is representation the same as reality? Is quantum theory lost in math, as Sabine Hossenfelder says? Is there a place for a sort of nostalgic realism? A way out of the complexity and open-endedness of the Many Worlds interpretation and such?
While Sean Carroll promotes an Austere Quantum Mechanics, this article (below) pitches a simpler foundational model – a plainer, more comprehensible connection to laws of motion for ordinary objects. But is it coherent?
In prior posts and comments, I’ve discussed the debate over the physical interpretation of the wave function – “the central mathematical object used in quantum mechanics” [1]. The connection of the wave function with reality: whether part of an effective theory which mathematically models (approximates) the way the universe works; or as something which directly reflects those workings. The epistemic (“bookkeeping”) vs. ontic viewpoints.
Are mathematical models free of any reduction in the nature of physical reality? Must the wave function be reified?
This article even references Sean Carroll. And gets tangled up in entanglement.
• Institute of Art and Ideas > “The quantum wave function isn’t real” by Eddy Keming Chen, Assistant Professor of Philosophy, University of California, San Diego, fellow of The John Bell Institute for the Foundations of Physics, recipient of the 2021 Karl Popper prize (April 29, 2022)
Here’re my bullets for how the article “reconsider[s] the realist interpretations of the wave function.”
• “The space on which the wave function is defined” entails extremely high dimensions – about 10^80 (huh?).
• As a real physical object, the wave function “leads to a surprising kind of holism” related to quantum entanglement – emergent group properties are disconnected from those of individual particles. That is, “most subsystems cannot be described by wave functions because of the phenomenon of quantum entanglement” (huh?).
• “Realist interpretations of the wave function seem to be in tension with Einstein’s relativity theory” via quantum entanglement’s ambiguous “way of slicing spacetime into space and time.”
Chen then touches on information theory, the density matrix, emergent patterns, the proper conceptual “zoom” level for physical laws, and the arrow of time.
According to the Past Hypothesis, “the universe started in a special state of very low entropy, at or near the Big Bang.” Which leads to “an attractive alternative to realist interpretations of the wave function.”
Enter an idea pitched as the “Wentaculus,” which “reduces the types of randomness in the world.” And in which the wave function is one of “a collection of many different hypothetical wave functions.”
While it includes homages to the historical debate, I find Chen’s article too abstract. As noted by a commenter, the “article is not written clearly enough to even form an opinion about it.”
There’s a too casual use of the term particle (and no references to quantum field theory). Presentationally, key points feel blurred. There’re no visualizations of perspectives or of indicated puzzles.
I do not see how this article advances discussion of the reality of the wave function.
Notes
[1] See related posts and these comments, for example:
• Quantum reality, quantum worlds – new book explores quantum foundations > December 9, 2019 and January 8, 2021.
• Quantum mechanics math basics – tasting the notation > July 21, 2020
[2] As mentioned above, Philip Ball notes in the chapter “There is no other ‘quantum’ you” of his book:
Related posts
• Whence the arrow of time?
• Quantum mechanics math basics – tasting the notation
• Cosmological fact and fiction
• A universe without math?
• Effective theory
This podcast with Sean Carroll re the quest for quantum gravity (cf. his latest book) includes his perspective on the wave function.
• Quanta Magazine > “Where Do Space, Time and Gravity Come From?” by Steven Strogatz (May 4, 2022) – my note from podcast transcript.
The wavefunction – “I think of the wavefunction as the fundamental thing … that’s what exists in reality.”
Entanglement between different pieces of the wavefunction – a relational nodal network in Hilbert space